Total Synthesis and the Creative Process:
An Interview with K.C. Nicolaou
Kyriacos C. Nicolaou, founding chairman of The Scripps
Research Institute (TSRI) Department of Chemistry and L.S.
Skaggs Professor of Chemical Biology at The Skaggs Institute
for Chemical Biology at TSRI, recently spoke with Jason Bardi
of News&Views. Nicoloau described his research, his approach
to training graduate students, and the field that builds complex
molecules from simpler ones-total synthesis.
Bardi: You have said that total synthesis demands those
same traits from a chemist as the creative process demands
from an artist. Can you explain this?
Nicolaou: Its rather complicated to even define
art, science, and technology. There is a triangle of art,
which is the pursuit of something new, usually associated
with esthetics; science, the pursuit of something new, perhaps
the understanding of nature; and technology, the application
of science.
Those of us who practice total synthesis like to think of
ourselves both as scientists and as artists. The molecules
that were dealing with in chemistry have dimensions,
geometries, and symmetries that are esthetically pleasing
and, perhaps, artistic. In our business, we design molecules
all the time, exercising artistic taste. We also exercise
artistic taste in the way that we combine chemical reactions
to arrive at a strategy that will lead to the target molecule.
A [composer] combines notes to make a symphony. In a similar
way, a synthetic chemist combines chemical maneuvers to make
a molecule. That sequence can be appreciated from an artistic
point of view. You have to understand the chemistry, of course,
to appreciate that.
Also, I can compare the art of the synthesis of a target
molecule to the game of chess. If you watch master chess players
and you follow their moves in getting to the king, you can
appreciate their mastery of the art, their ingenuity, their
cleverness. In the same way, if we are making a molecule,
we have to go step-wise from the material we can buy, from
petroleum, or from crops, or from something we can extractand
we have to make maybe 50 moves before arriving at a very complicated
product, such as taxol or vancomycin.
Along the way, we face an opponent. It is an invisible opponent.
Nature. We are designing a particular step-maybe the
35th stepand we dont know if that step will be
allowed by the natural laws. We have to experiment to see
if its going to work. We anticipate all our moves, and
we write them down on a piece of paper. But it never works
that way. Nature always counters us.
In the laboratory, we always come up against obstacles to
overcome, often not anticipated. These obstacles are actually
blessings in disguise, because [they force us to] rise to
a higher level of ingenuity and create new chemistry to solve
that particular problem.
So you come up with new chemistry?
Absolutely. We invent new chemistry. Most important are
the things you discover along the way that remain for everyone
to use in the laboratory.
If you practice total synthesis, you are bound to sharpen
the tool of organic synthesis. And thats very necessary
today with the human genome deciphered because we need to
have the capability of making more molecules faster than before.
We need to have more complicated molecules. We need to make
them more efficiently. We need to make them in a benign chemical
way so that we dont pollute the environment. And total
synthesis continues to be the engine that drives organic synthesis
forward, and therefore I think it is still a flourishing,
exciting field of investigation.
How is the human genome initiative going to affect total
synthesis, andlikewisehow will total synthesis
be used as a tool in genomics?
The importance of [total synthesis] is finding ways to increase
the pace of producing compounds for biological screening.
And this is what the human genome demandsmatching all
those discoveries in biology with advances in synthetic chemistry
that will produce the ligands that we need to modulate the
function of the genes.
Eighty percent of the medicines we have in the pharmacy
today are small organic molecules, which go into the body,
bind certain proteins, and modulate their effect. Now that
we have so many more biological targets, we need even more
small molecules. We need libraries of small molecules from
which we can select the ones that bind to the proteins, the
ones that have the right pharmacological profile so that they
can become medicines. The bottom line is synthesizing a lot
of compounds and testing them against a biological target.
We have to synthesize many compounds to find a few that
bind. And even if we find a few that bind, that doesnt
mean they are drugs. We have to fine-tune their structure
to make them have the right propertiesbioavailability,
stability, toxicity, all those things.
Organic synthesis is behind medicinal chemistry, and total
synthesis is a branch of organic synthesis. I call it the
engine that drives organic synthesis forward, because total
synthesis deals with the ultimate challenges of organic synthesisthe
most complicated molecules that nature has ever made are in
front of the synthetic chemist.
[Total synthesis] also provides a test for the state of
the art of chemical synthesis. If you can make taxol, then
it means that your state of the art is very powerful. Ten
years from now, we need to make much more complicated molecules
than taxol. And at that time, Im sure well be
able to do it, because well have more refined tools.
1 | 2 | 3
|
|
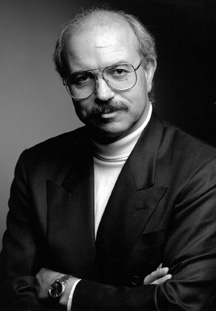
"A composer combines notes to make a
symphony," says Professor K.C. Nicolaou. "In a similar way,
a synthetic chemist combines chemical maneuvers to make a
molecule."
Persist
and withdraw when you are faced with difficulties, redesign
the strategies, and try again.
K.C.
Nicolaou
|