Total Synthesis and the Creative Process:
An Interview with K.C. Nicolaou
Kyriacos C. Nicolaou, founding chairman of The Scripps Research Institute
(TSRI) Department of Chemistry and L.S. Skaggs Professor of Chemical Biology
at The Skaggs Institute for Chemical Biology at TSRI, recently spoke with
Jason Bardi of News&Views. Nicoloau described his research, his approach
to training graduate students, and the field that builds complex molecules
from simpler ones-total synthesis.
Bardi: You have said that total synthesis demands those same traits
from a chemist as the creative process demands from an artist. Can you
explain this?
Nicolaou: Its rather complicated to even define art, science,
and technology. There is a triangle of art, which is the pursuit of something
new, usually associated with esthetics; science, the pursuit of something
new, perhaps the understanding of nature; and technology, the application
of science.
Those of us who practice total synthesis like to think of ourselves
both as scientists and as artists. The molecules that were dealing
with in chemistry have dimensions, geometries, and symmetries that are
esthetically pleasing and, perhaps, artistic. In our business, we design
molecules all the time, exercising artistic taste. We also exercise artistic
taste in the way that we combine chemical reactions to arrive at a strategy
that will lead to the target molecule.
A [composer] combines notes to make a symphony. In a similar way, a
synthetic chemist combines chemical maneuvers to make a molecule. That
sequence can be appreciated from an artistic point of view. You have to
understand the chemistry, of course, to appreciate that.
Also, I can compare the art of the synthesis of a target molecule to
the game of chess. If you watch master chess players and you follow their
moves in getting to the king, you can appreciate their mastery of the
art, their ingenuity, their cleverness. In the same way, if we are making
a molecule, we have to go step-wise from the material we can buy, from
petroleum, or from crops, or from something we can extractand we
have to make maybe 50 moves before arriving at a very complicated product,
such as taxol or vancomycin.
Along the way, we face an opponent. It is an invisible opponent. Nature.
We are designing a particular step-maybe the 35th stepand
we dont know if that step will be allowed by the natural laws. We
have to experiment to see if its going to work. We anticipate all
our moves, and we write them down on a piece of paper. But it never works
that way. Nature always counters us.
In the laboratory, we always come up against obstacles to overcome,
often not anticipated. These obstacles are actually blessings in disguise,
because [they force us to] rise to a higher level of ingenuity and create
new chemistry to solve that particular problem.
So you come up with new chemistry?
Absolutely. We invent new chemistry. Most important are the things you
discover along the way that remain for everyone to use in the laboratory.
If you practice total synthesis, you are bound to sharpen the tool of
organic synthesis. And thats very necessary today with the human
genome deciphered because we need to have the capability of making more
molecules faster than before. We need to have more complicated molecules.
We need to make them more efficiently. We need to make them in a benign
chemical way so that we dont pollute the environment. And total
synthesis continues to be the engine that drives organic synthesis forward,
and therefore I think it is still a flourishing, exciting field of investigation.
How is the human genome initiative going to affect total synthesis,
andlikewisehow will total synthesis be used as a tool in genomics?
The importance of [total synthesis] is finding ways to increase the
pace of producing compounds for biological screening. And this is what
the human genome demandsmatching all those discoveries in biology
with advances in synthetic chemistry that will produce the ligands that
we need to modulate the function of the genes.
Eighty percent of the medicines we have in the pharmacy today are small
organic molecules, which go into the body, bind certain proteins, and
modulate their effect. Now that we have so many more biological targets,
we need even more small molecules. We need libraries of small molecules
from which we can select the ones that bind to the proteins, the ones
that have the right pharmacological profile so that they can become medicines.
The bottom line is synthesizing a lot of compounds and testing them against
a biological target.
We have to synthesize many compounds to find a few that bind. And even
if we find a few that bind, that doesnt mean they are drugs. We
have to fine-tune their structure to make them have the right propertiesbioavailability,
stability, toxicity, all those things.
Organic synthesis is behind medicinal chemistry, and total synthesis
is a branch of organic synthesis. I call it the engine that drives organic
synthesis forward, because total synthesis deals with the ultimate challenges
of organic synthesisthe most complicated molecules that nature has
ever made are in front of the synthetic chemist.
[Total synthesis] also provides a test for the state of the art of chemical
synthesis. If you can make taxol, then it means that your state of the
art is very powerful. Ten years from now, we need to make much more complicated
molecules than taxol. And at that time, Im sure well be able
to do it, because well have more refined tools.
This field is augmented by the discovery of new structures in nature.
Nature has not revealed all its secrets to us yet. In the forest, there
are so many hidden compoundswe dont know their structure and
their use. In the soil, where the bacteria and the fungi live, there are
also many hidden compounds. The ocean is full of organisms we have not
explored. Im sure that it is rich in new molecular structures which
will come to our attention sooner or later. They will challenge synthetic
chemists to invent new methodologies to synthesize these new types of
structures.
I see a great future for the art and science of total synthesis. And,
in addition to that, it is a great area to train graduate students and
post-docs because it puts in front of them severe challenges in which
they have to bring to bear all the tools of synthetic chemistry. They
have to sharpen their minds and become practitioners in the art of making
these molecules. There is a great need for this kind of training todayin
fact, there is a shortage in manpower in this field. We do not have enough
students and people in the industry will tell you the same thing.
Whats a typical project for graduate students?
Typical project? I can mention famous compounds like taxol, brevetoxin,
vancomycinthese are very famous moleculesthe CP molecules
we have synthesized recently, or the epothilones which are extremely powerful
anti-tumor agents. There are many. Thats what makes it so exciting,
you see. The field is very vibrant, very exciting, and we are very fortunate
to have a great number of talented students and post-docs here.
The practitioners of total synthesis exercise taste in selecting these
targets. We look at the molecular architecture, [for] something that looks
completely novel. Structures which Ive seen for 25 years dont
excite me any more, but if I see something entirely new, unusual architecturally,
then Im attracted to it.
I also look for important biological activity in looking for a target.
Taxol is an anti-cancer agent, vancomycin is an antibiotic. Thats
an additional advantage because at the end of the road you know you are
going to have a biological twist to your project. Combining chemistry
and biology makes the project more worthwhile, more exciting, and more
fulfilling for the student. They get training in both fields at the same
time.
How do students deal with all the problems that can arise in a complicated,
multiple-step synthesis?
Typically when they come into the group, we have discussions about the
various projects that are ongoing. Sometimes they can join an ongoing
project, [and] sometimes we pick new targets from the literature. Then
we make a plan. I do encourage the students to participate in this planning,
because that is a very important aspect of their education. We have to
teach them how to think creatively and design their own synthetic strategies,
because this is what is going to make them full and complete scientists
who stand on their own feet. And thats very attractive to them as
well. They dont want to be a pair of hands following the instructions
of the professor. They want to be partners all the way from the beginning
so they can exercise their creativity and sharpen their skills intellectually
as well as experimentally.
There are many, many problems awaiting us along the path. We dont
worry about that. Thats a given, and we know thats part of
the excitement. We make as good a plan as we can, given the knowledge
that we have, and dont worry about the rest.
I tell the students, Just get in the lab, we have a very good
plan to follow, and things are going to crystallize sooner or later. Persist
and withdraw when you are faced with difficulties, redesign the strategies,
and try again.
[In this field], you are going to fall on the ground many times. This
practice requires stamina, requires character. Its a real test of
character, whether you can withstand these failures, one right after another.
But thats what makes a skillful professional. Whether its
in business, in science, or in any endeavor. You have to be able to face
difficulties and develop the abilities and methods to face these difficultiesto
stand on your feet and try and try again. Sooner or later this seemingly
unsolvable problem will melt in front of this human persistence and innovation.
And as youre going along the path, you discover things that you
never expectedcompletely by serendipity. Sometimes its like
a hidden vein in the ground, which gives you gold or oil. [This vein]
yields many things to figure out and explore. Of course, you have to be
alert and astute to recognize an important discovery like this when it
comes along.
Can you give me an example of such serendipitous discoveries?
Well, a very good example is one of our recent projects in the CP molecules.
These CP molecules were really diabolical in their molecular architecture.
They posed untold problems and challenges before they finally succumbed
to the assault by the students who were involved. Along the way, we encountered
an unexpected result, which did not help us solve the problem and yet
gave us a gold mine of new synthetic methods to make heterocycling molecules.
They are the ideal molecule for biological screening because they have
the ability to bind to proteins. So out of that serendipitous discovery,
which we made a couple of years ago, we probably have a dozen publications
that describe new synthetic methodology that can be applied to combinatorial
chemistry to give us compound libraries for biological evaluation.
What do these combinatorial libraries look like? These 10,000 different
compounds?
Yes, we have published papers on the 10,000 member libraries, as you
know. What inspired us there was, in looking in the literature at natural
products for medicinal chemistry, we recognized that there were a great
number of naturally occurring compounds and medicinal compounds [with
common structures]. And they were endowed with a variety of biological
effects, including anti-cancer, antibiotic, antiviral, enzyme inhibitory
and so on.
So immediately, we felt that instead of waiting for people to go and
collect these compounds from nature, one at a time, we could design a
strategy in which we could produce them in the laboratory in large numbers,
and make relatives of these compounds, analogues of these compounds. We
call these libraries.
The universe of molecular diversity, as we call it, is hugeessentially
unlimited. The wise thing to do is to design sub-libraries. From this
huge universe of molecular diversity, we design a sub-library, say, looking
like vancomycin, or the epothilones, or like nothing youve seen
beforesomething that youve just imaginedbut within the
domain of structural variation that you can make through the same synthetic
technology. Yet you can have a variation of 10,000 compounds. Then once
youve done this library, you can start over from scratch. Totally
different structures, again, but within a framework, a scaffold.
In the last decade, you and your lab have synthesized rapamycin,
vancomycin, taxol, brevetoxin, epothilones...
And calicheamicin. Dont forget calicheamicin. Thats an important
one. 1992.
This compound came out of a rock. It was collected at Waco, Texas, in
1987 by a touring scientist. He picked up this rock thinking, "Maybe theres
some bacteria or fungi living inside." So he brought this rock to the
laboratory and grew a culture. Inside this culture they discovered a compound
called calicheamicin. A phenomenally active compound, extremely active
against tumor cellsit binds to DNA, chops it into pieces, and kills
the tumor cells.
It was too toxic to be used as a medicine, but the structure was so
beautiful, so unusual, and unprecedentedit looked impossible to
synthesize. We had the courage to try to make this compound, and we synthesized
it in 1992. Then, after several years, people in industry attached this
compound to antibodies so they were able to deliver it to certain cancer
types selectively without the side effects of the very toxic compound.
And its now in the clinic. Its been approved by the FDA. Certain
types of lymphomas, I believe, can be attacked by conjugates of the calicheamicin
with the antibodies. The story of this compound is fascinating, and Im
very proud of it.
So whats next?
Thats a good question. Everybodys been asking me the same
question for the last 10 years. "Whats next?" Its like the
stock market: what should we buy next? [LAUGHS]. If I knew, Id be
a much more famous chemist than I am today.
Let me ask you a more general question then. In looking to the 21st
century, what are the big synthetic targets for you and for others in
the field?
Well, I think the best targets in the field are going to reveal themselves
to us in the future. We have only just touched the tip of the iceberg
in terms of molecular diversity from nature. A lot of the structures that
we have synthesized were not known to us 10 years ago. I strongly believe
that we are going to see a lot of new structures creeping into the literature
with exciting biological activities that will keep us busy.
I consider myself very fortunate to be in such an exciting field. Its
not necessarily that we will discover the miracle drugs of tomorrow, but
the basic science that we develop is the kind that is used in the pharmaceutical
and biotechnology industries to invent and discover the new generation
of medicines. The training that we provide to the students is very instrumental
for that. Its basically the collection of the accomplishments of
my students that I am most proud of. And instilling in these students
the habit of rational and deep thinking required by such projects, an
invaluable companion for their future careers.
Is there anything else?
Its very important to give credit to all my teammy students,
my staff, Vicky Nielsen, Janise Petrey, my colleagues in the chemistry
department, and our administration here, especially Richard Lerner [president
of TSRI] for his visionary moves and his generosity and support over the
years. We couldnt have done what we have done in the chemistry department
without his support.
Go back to News & Views Index
|
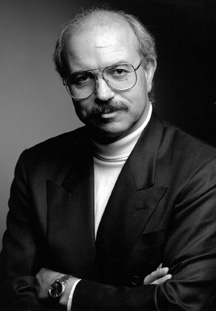
"A composer combines notes to make a symphony,"
says Professor K.C. Nicolaou. "In a similar way, a synthetic chemist combines
chemical maneuvers to make a molecule."
Persist and withdraw when you are faced with difficulties, redesign the
strategies, and try again.
K.C.
Nicolaou
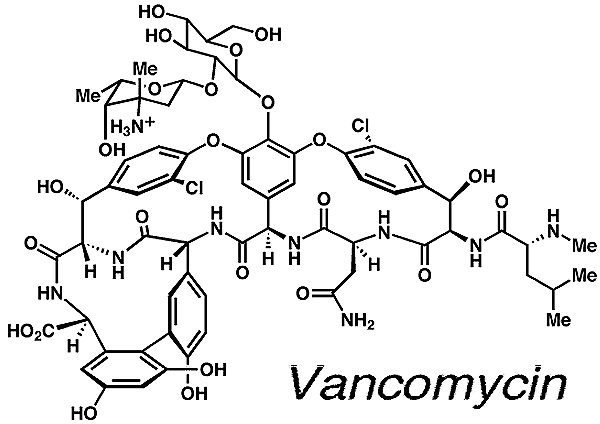
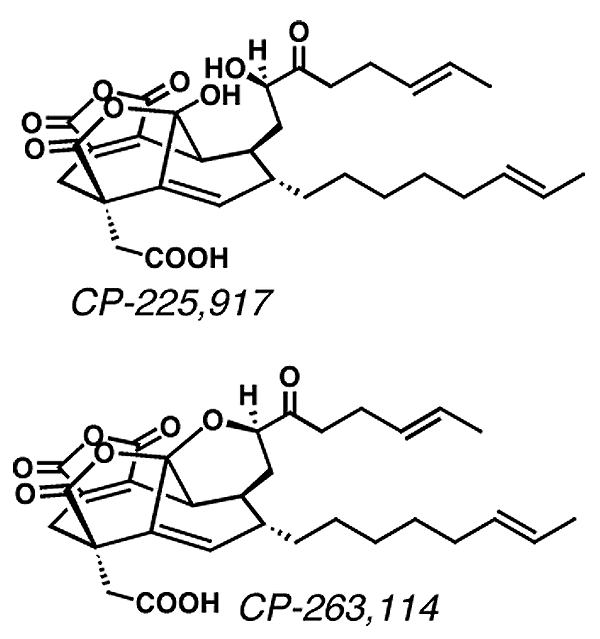
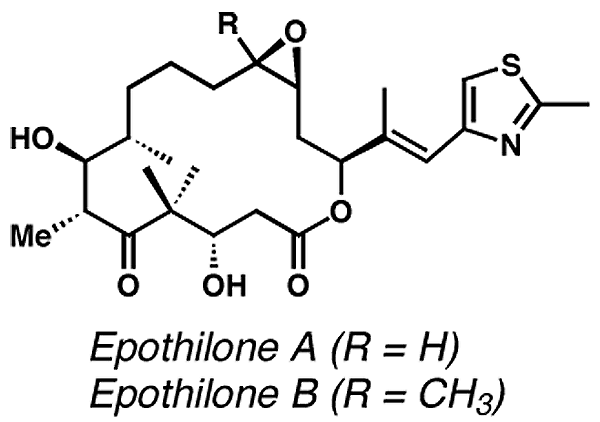
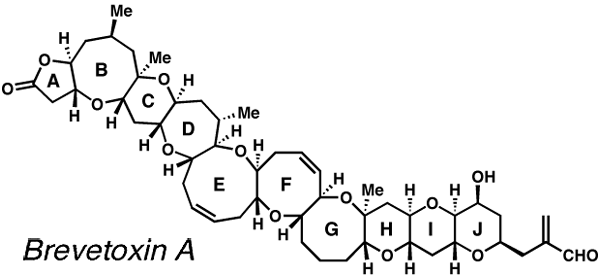
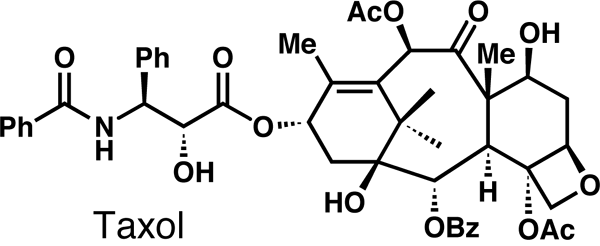
Some of the molecules that the Nicolaou lab has
synthesized include vancomycin, CP-225,917, CP-263,114, epothilone A and
B, brevetoxin A, and taxol.
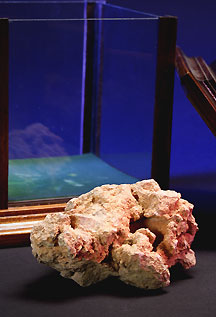
A rock, above, collected by a touring scientist
in Waco, Texas, yielded calicheamicin, below, a compound extremely active
against tumor cells. "We have only just touched the tip of the iceberg
in terms of molecular diversity from nature," says Nicolaou.
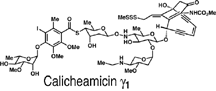
|