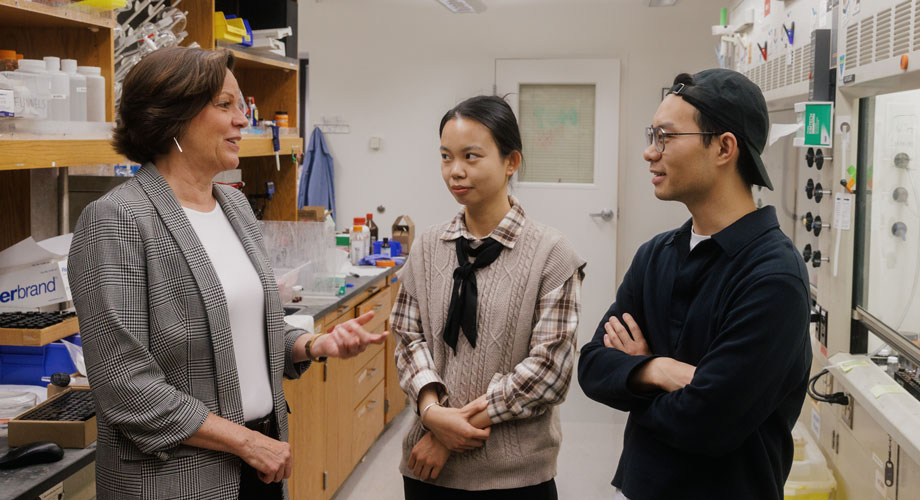
Donna Blackmond, PhD, senior author of both studies; Jinhan Yu, first author of the PNAS study; and Min Deng, PhD, first author of the Nature study. Credit: Scripps Research
How molecular “handedness” emerged in early biology
Scripps Research chemists fill a major gap in origin-of-life theories.
February 28, 2024
LA JOLLA, CA—Molecules often have a structural asymmetry called chirality, which means they can appear in alternative, mirror-image versions, akin to the left and right versions of human hands. One of the great mysteries about the origins of life on Earth is that virtually all of the fundamental molecules of biology, such as the building blocks of proteins and DNA, appear in just one chiral form.
Scripps Research chemists, in two high-profile studies, have now proposed an elegant solution to this mystery, showing how this single-handedness or “homochirality” could have become established in biology.
The studies were published in the Proceedings of the National Academy of Sciences on February 5, 2024, and in Nature on February 28, 2024. Together, they suggest that the emergence of homochirality was due largely to a chemistry phenomenon called kinetic resolution, in which one chiral form becomes more abundant than another due to faster production and/or slower depletion.
“There have been many proposals for how homochirality emerged in specific molecules—specific amino acids, for example—but we really have needed a more general theory,” says Donna Blackmond, PhD, professor and John C. Martin Chair in the Department of Chemistry at Scripps Research, who led both studies.
Graduate student Jinhan Yu and postdoctoral research associate Min Deng, PhD, were the first authors of the two studies.
The conundrum of homochirality
“Origin of life” chemistry has been a busy field for much of the past century. Its practitioners have discovered dozens of key reactions that plausibly occurred on the early, “prebiotic” Earth to produce the first DNAs, RNAs, sugars, amino acids and other molecules that sustain life. Missing from this body of work, however, has been a plausible prebiotic theory for the emergence of homochirality.
“There has been a tendency in the field to ignore the chirality issue when looking for plausible reactions that could have made the first biological molecules,” Blackmond says. “It’s frustrating, because without reactions that favor homochirality, we wouldn't have life.”
Ordinary chemical reactions that produce chiral molecules tend to yield equal (“racemic”) mixes of left- and right-handed forms. Outside of biology, this mixing typically doesn’t matter, as both forms usually have similar or identical properties. Within biology, though, as a consequence of extensive homochirality, it is commonly the case that only the left- or the right-handed form of a chiral molecule has useful properties—the other may be inert or even toxic. Thus, cells often guide reactions to yield specific chiral forms, using highly evolved enzymes.
The prebiotic Earth would not have had such enzymes, though—so how did homochirality ever arise?
A paradoxical result
In their study in Proceedings of the National Academy of Sciences, Blackmond and her team addressed this problem for amino acids. These small organic molecules are used as building blocks for proteins by all living things on Earth, but exist in biology in just the left-handed chiral form.
The researchers specifically sought to reproduce homochirality in a central process in amino acid production called transamination, by using a relatively simple, plausibly prebiotic chemistry that excludes complex enzymes.
In early tests, the team’s experimental reaction worked, and yielded amino acids that were enriched for one chiral form versus the other. The problem was that the favored form was the right-handed form—the one that biology doesn’t use.
“We were stuck for a while, but then the light bulb went on—we realized we could do part of the reaction in reverse,” Blackmond says.
When they did that, the reaction no longer preferentially made right-handed amino acids. In a striking example of kinetic resolution, it instead preferentially consumed and depleted the right-handed versions—leaving more of the desired left-handed amino acids. It thus served as a plausible route to homochirality for amino acids used in living cells.
Tying it all together
For the Nature study, the chemists explored a simple reaction with which amino acids in the earliest life forms might have been linked together into the first short proteins (also known as peptides). The reaction had been published earlier by another researcher, but had never been investigated for its ability to produce homochiral peptides from racemic or near-racemic mixes of amino acids.
Once again, the chemists ran into what seemed to be an insurmountable obstacle: They discovered that in forming peptide chains of amino acids, the reaction worked faster for linkages of left-handed with right-handed amino acids—the opposite of the desired homochiral peptides.
Still, the team persevered. Ultimately, they discovered that when one type of amino acid in the starting pool of amino acids had even a moderate dominance of the left-handed form—as their other study made plausible—the faster reaction rate for left-handed-to-right-handed linkages preferentially depleted right-handed amino acids, leaving an ever-greater concentration of left-handed ones. Additionally, the left-right-left-right peptides had a stronger tendency to clump together and fall out of solution as solids. These kinetic resolution-related phenomena thus ended up yielding a surprisingly pure solution of almost fully left-handed peptides.
To Blackmond, the seemingly paradoxical mechanisms uncovered in these studies offer the first convincing and broad explanation for the emergence of homochirality—an explanation that probably works not only for amino acids, she says, but also for other fundamental molecules of biology such as DNA and RNA.
“Prebiotic access to enantioenriched amino acids via peptide-mediated transamination reactions” was co-authored by Jinhan Yu, Andrea Darú, Min Deng and Donna Blackmond.
“Symmetry breaking and chiral amplification in prebiotic ligation reactions” was co-authored by Min Deng, Jinhan Yu and Donna Blackmond.
Funding for both studies was provided by the Simons Foundation through the Simons Collaboration on the Origins of Life (SCOL 287625), and through the John C. Martin Endowed Chair in Chemistry at Scripps Research.
For more information, contact press@scripps.edu