Research Description
Introduction
Understanding the brain – how it develops, carries out complex tasks, and is affected by diseases – is the overall goal of our research. This is being pursued through two scientific areas that have both basic science and therapeutic implications: 1) lysophospholipid (LP) receptor signaling, involving small lipids that act as extracellular chemical signals; and 2) genomic mosaicism amongst single brain cells – especially neurons – whereby cells from the same individual can vary at the genomic level. Within these areas, we have placed a particular emphasis on translating the basic science research into an understanding and potential treatment of brain diseases, with current efforts aimed at hydrocephalus, Multiple Sclerosis (MS), Alzheimer’s Disease, and other neurodegenerative diseases. The entry of fingolimod – a compound that acts through a subgroup of lysophospholipid receptors – into the clinical treatment of MS, underscores the real-life relevance and future potential of these lines of research.
An overview of Dr. Chun and the lab's research can be found in the Fall 2014 publication, Scripps Discovers, volume 10, number 4. Download here.
Lysophospholipid Receptor Signaling
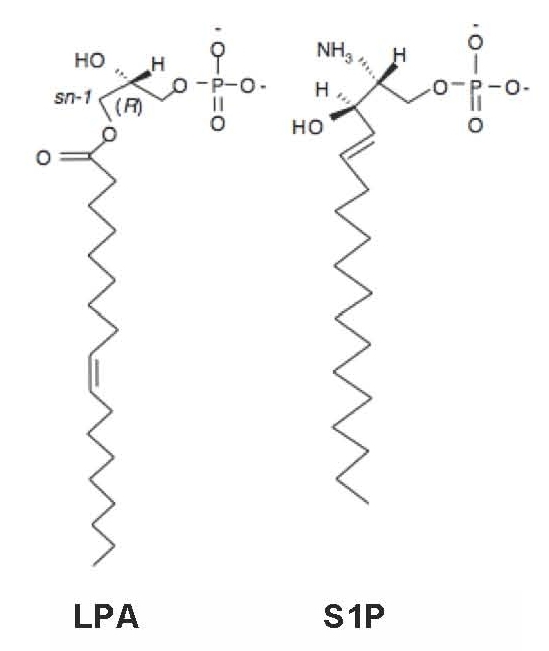
Lysophospholipids are simple phospholipids commonly derived from cell membranes. They contain a glycerophosphate or sphingoid backbone and a linked, single aliphatic chain of varied length and saturation. Two of the best-studied forms of lysophospholipids are the glycerophospholipid called lysophosphatidic acid (LPA) and the sphingolipid known as sphingosine 1-phosphate (S1P), which are both part of a larger group of lysophospholipids.
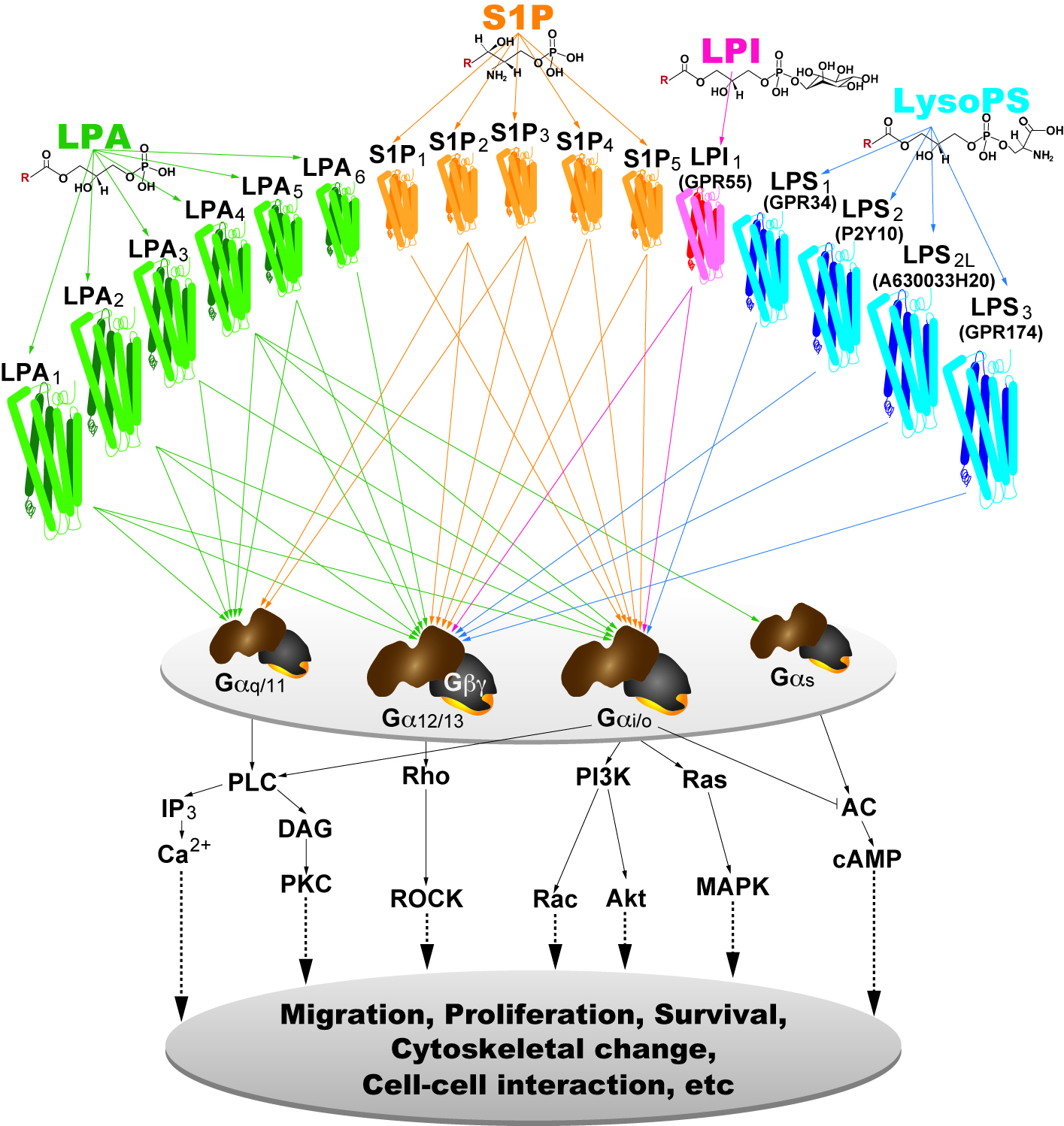
It is now clear from the work of many labs that the most important actions of lysophospholipids are mediated by receptors on the cell surface. These are cognate, 7-transmembrane, G protein-coupled receptors (GPCRs) that now number at least 15: six LPA receptors, five S1P receptors, three likely lysophosphatidyl serine (LPS) receptors, and one lysophosphatidyl inositol (LPI) receptor . The creation of mice null for single and multiple receptor subtypes, including the generation of cell-specific null receptor mutants, is being used to determine receptor selectivity of physiological and pathophysiological functions. This approach, in combination with pharmacological tools, has illustrated the wide-ranging effects of LP receptor signaling – most organ systems and cell types during development, adult life, and in disease are affected by LP receptor signaling. Ongoing research using a range of cutting-edge biophysical technologies is aimed at identifying new lysophospholipid receptors and/or interactions, determining new receptor-mediated physiological and pathophysiological functions affecting the brain, and initiating related drug development efforts.
Hydrocephalus
A key example of the relevance of lysophospholipid signaling in understanding brain disease mechanisms is seen in LPA’s roles in hydrocephalus, one of the most common neurological diseases of newborns and young children.
Hydrocephalus has historically been thought to be a “plumbing” problem, with the blockage of fluid outflow and the over-accumulation of cerebral spinal fluid in the brain. Currently, only surgical, palliative treatments exist for treating hydrocephalus. During studies on the effects of LPA signaling for embryonic cerebral cortical development, we found that over-activation of LPA signaling in neural progenitor cells, as can be produced by LPA during hemorrhage, can mechanistically account for initiating post-hemorrhagic hydrocephalus. Critically, disease initiation involves defined LPA receptors, raising the possibility of pharmacologically interrupting the progression of hydrocephalus through the use of LPA receptor antagonists. We are currently delineating the cellular and molecular pathways (with an emphasis on identifying medically tractable pathways) involved in hydrocephalus.
Multiple Sclerosis (MS)
MS is the most common cause of neurological disability in young adults. It is a disease in which the body’s immune system attacks the brain and it produces a range of neurodegenerative symptoms that ultimately leads to death. Common treatments had historically involved shots or injections. In 2010, the FDA approved a compound known as fingolimod (commercial name Gilenya) that represented the first oral therapy for MS. Fingolimod was discovered in Japan during efforts to understand and modify chemicals produced by fungi; one species of fungus called Isaria sinclairii – a curious fungus that infects and grows out of the body of underground insects – led to the identification of fingolimod.
Fingolimod, once phosphorylated, is an S1P receptor modulator. Fingolimod is postulated to reduce the entry of pathogenic lymphocytes into the CNS by binding to S1P receptors on immune cells. In addition, recent data have identified direct CNS actions of S1P signaling in MS, particularly through the use of animal models like experimental autoimmune encephalomyelitis (EAE), in combination with genetically altered animals that lack receptors in brain cell sub-populations. These types of studies have identified astrocytes as key players in the actions of S1P signaling. Current studies are focused on understanding Fingolimod’s ability to prevent or repair damage caused by MS by directly affecting the brain. Genetically altered mice and cutting-edge cellular and molecular approaches are being used.
Genomic Mosaicism in the Nervous System
The human brain contains an enormously diverse repertoire of cells based on their appearance, molecular profile, and function. The vast majority of these cells arise before birth (e.g., neurons of the cerebral cortex) or soon after birth, thus “gifting” a person with neurons that will hopefully remain throughout life. Cells in the developing brain divide very rapidly. During cell division, DNA is replicated then distributed equally into each daughter cell. The cell packages DNA into chromosomes, discrete clumps of the genome that can be seen with a microscope and are characteristic in number or form for a given species. Human cells have 23 pairs of chromosomes (46 chromosomes of which two are sex chromosomes). Each chromosome contains hundreds of genes along with even more “non-coding” DNA that control the expression of all the components that make up cells, tissues, and organs of the body.
During studies of the brain, and genes that could affect DNA, we observed that sometimes the chromosomes are not equally distributed when cells in the developing brain divide. Unequal distribution of chromosomes result in cells that have less than or more than two copies of each chromosome: these are called aneuploid cells. Aneuploid cells are found outside of the brain, most notably in cancer cells, but were not believed to occur within the brain. Textbooks continue to teach the idea that every human cell must have 23 pairs of chromosomes – no more, no less – in order to function normally. Cells that have lost or gained chromosomes are presumed to either die or become cancerous. However, using a combination of spectral karyotyping (SKY), which “paints” chromosomes to allow their unambiguous detection, and fluorescence in situ hybridization (FISH), which utilizes labeled point-probes to identify discrete chromosomal loci in interphase cells, we have found that aneuploid cells are produced in high numbers in the developing brain – perhaps upwards of 40%. Although many of these cells do die, many of them also appear to survive to both influence development and to become part of the adult brain.
The existence of aneuploidy is also a clue that other processes affecting the genome are taking place, and research has revealed other forms of changes to the genome take place in brain cells (these changes are collectively known as genetic mosaicism). Additional forms of genetic mosaicism, such as increased gains in total DNA, showed an interesting neuroanatomical segregation. Notably, the prefronal cortex of the human brain displays significant net DNA gains, but other areas, such as the cerebellum, do not. We speculate that aneuploidy and other forms of variation, collectively known as DNA content variation, contribute to the diversity of brain cells, allowing the brain to perform highly complex functions such as learning, memory, and creative thought. DNA content variation may also contribute to the spectrum of human uniqueness, ranging from genius to madness. In addition, the many diseases afflicting the human brain are notable for being overwhelmingly sporadic in nature. Many explanations for these sporadic diseases rely purely on classical genetics, where families with a “bad” gene pass the gene on to their affected children, and to every cell of that child. However, classical genetics only reliably describes a small portion of patients. We are currently using modern techniques to understand how the genome might “sporadically” change, what produces this change, and the consequences of such a change in the normal as well as diseased brains.
For an overview of this work, please see "Rewiring the brain" courtesy of International Innovation - a leading scientific dissemination service.
NIH Funding
- U01 MH098977 (Kun Zhang, PI, UCSD; Jerold Chun, PI, TSRI), "Single-cell sequencing and in situ mapping of RNA transcripts in human brains"
- R01 AA021402 (Jerold Chun, PI), "Mechanisms and consequences of CNS aneuploidies altered by fetal alcohol exposure"
- R01 NS084398 (Jerold Chun, PI), "Prenatal blood-borne lipids in post-hemorrhagic hydrocephalus"
- UL1 TR001114 (Jerold Chun, Pilot Award PI), "Mechanisms and consequences of mosaic APP amplification in sporadic AD neurons"