A Primer on the NMR of Biological Macromolecules
Nuclear magnetic resonance (NMR) refers to the ability of
atomic nuclei to reorient in a magnetic field when exposed
to radiation of a particular "resonant" frequency in the radio
band.
Certain atomic nuclei ("NMR isotopes") contain charged particles
with spin, which according to Maxwell's equations, induces
a magnetic field. Though small, the magnetic "moments" of
these nuclei makes them sensitive to an external magnetic
field. In an NMR magnet, the nuclei act like tiny bar magnets
and tend to align themselves preferentially in a particular
configuration, while also undergoing spinning motions similar
to the gyroscopic precessions of bicycle wheels or spinning
tops under an external torque.
Any fluctuating magnetic field orthogonal to that of the
NMR magnet will perturb the alignment of the nuclear magnetic
moments away from the equilibrium configuration, but only
if the frequency of the fluctuating field is precisely equal
to the precession frequencies of the nuclear magnetic moments.
These are called the resonant, or Larmor, frequencies and
are proportional to the field strength of the NMR magnet.
The Scripps Research Institute's (TSRI's) new 21 tesla magnet,
for instance, causes protons to precess at 900 MHz. Movement
of atomic nuclei in the NMR as they go in and out of resonance
causes small but measurable induced voltages, and it is this
signal which is being measured in the NMR experiment.
An NMR spectrometer will scan a broad range of radio frequencies
and record all the resonances as a spectrum. Atoms like 1H,
13C, or 15N, which are ubiquitous in
proteins and nucleic acids, have a nuclear spin and give rise
to NMR signals, whereas atoms like 12C and 16O
have no nuclear spin and therefore no signal. Different spectra
can be taken with molecules that have been selectively labeled
with isotopes that have or do not have a spin.
In an NMR experiment, a sample in a glass tube is inserted
into the magnet, and the resonant responses of the atoms in
the sample over a range of frequencies are recorded. These
responses are influenced by the shape of the molecule in which
the atoms resideby their proximity to other atoms in
the molecule. An NMR spectrum is unique for a particular molecule,
and the structure of a molecule can be determined from its
spectrum.
There is no question that NMR is one of the fundamental
techniques in chemistry and biology today.
In fact, three Nobel prizes have now been awarded for work
on the technique. The 1952 Nobel Prize in Physics went to
two physicists, Edward Mills Purcell at Harvard University
and Felix Bloch at Stanford University, who discovered the
NMR effect independently in 1946. The 1991 Nobel Prize in
Chemistry was awarded to Richard R. Ernst at ETH in Zürich
for "the development of the methodology of high resolution
nuclear magnetic resonance (NMR) spectroscopy."
Most recently, the 2002 Nobel Prize in Chemistry was awarded
to TSRI's Kurt Wüthrich "for his development of nuclear
magnetic resonance spectroscopy for determining the three-dimensional
structure of biological macromolecules in solution."
|
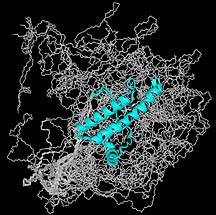
NMR structure of the bovine prion protein,
solved by TSRI investigator Kurt Wüthrich. For more images,
see the Wüthrich
home page.
|