(Page 2 of 2)
The Huge Advantage of TROSY
A major breakthrough has come in the form of a new technique
that is sure to take advantage of the size of the new 900
MHz instrument. This technique is called transverse relaxation
optimized spectroscopy (TROSY).
TROSY is a technique that suppresses the transverse nuclear
spin relaxationa quantity that causes the deterioration
of NMR spectra for larger molecular structures. "What TROSY
lets you do is work on really big systems," says Molecular
Biology Professor Jamie Williamson.
Using TROSY, the upper size limit for molecular structures
that can be studied with NMR can be extended to include those
that are hundreds of kD in size. "This is one of the things
we are going to do with the 900," says John Chung, director
of TSRI's Biomolecular NMR facility.
The optimal frequency for the TROSY effect is calculated
to be near one GHz, and since 900 MHz is closer to this than
any instrument ever before, Wright, Dyson, Williamson and
their colleagues believe that they will be able to effectively
apply TROSY to very high molecular weight systems on the new
instrument.
"Up to this point we have been limited to about 30 kD,"
says Williamson, whose research involves looking at protein/RNA
complexes such as Rev and Tat protein bound to HIV RNA. Another
set of structures he studies are intermediates in the assembly
of the bacterial ribosome, which when fully assembled is about
2.7 million daltons. "Now we can ask how do these pieces that
we look at look in the context of the larger assembly," he
says.
Moreover, Kurt Wüthrich, who was the first to recognize
and exploit TROSY at ultra-high magnetic field strengths with
his colleagues in Switzerland, is coming to TSRI this year
as a visiting professor, while maintaining his position at
ETH in Zurich.
"This is an NMR Mecca right nowthis is where it's
all happening," says Wright. "And now, with Wüthrich
coming, it's going to be even more of a Mecca."
NMRAn Old Friend to Modern Biology
Discovered in 1946 by independent groups at Stanford and
Harvard Universities, NMR refers to the ability of atomic
nuclei to reorient themselves in a magnetic field when exposed
to radiation of a particular "resonant" frequency in the radio
band.
Atomic nuclei contain charged particles with spin, which
according to Maxwell's equations, induces a magnetic field.
Though small, the magnetic "moments" of these nuclei makes
them sensitive to an external magnetic field. In an NMR magnet,
the nuclei act like tiny bar magnets and tend to align themselves
preferentially in a particular configuration, while also undergoing
spinning motions similar to the gyroscopic precessions of
bicycle wheels or spinning tops under an external torque.
Any fluctuating magnetic field orthogonal to that of the
NMR magnet will perturb the alignment of the nuclear magnetic
moments away from the equilibrium configuration, but only
if the frequency of the fluctuating field is precisely equal
to the precession frequencies of the nuclear magnetic moments.
These are called the resonant, or Larmor, frequencies and
are proportional to the field strength of the NMR magnet.
TSRI's new 21 tesla magnet, for instance, causes protons to
precess at precisely 900 MHz. Movement of atomic nuclei in
the NMR as they go in and out of resonance causes small but
measurable induced voltages, and it is this signal which is
being measure in the NMR experiment.
An NMR spectrometer will scan a broad range of radio frequencies
and record all the resonances as a spectrum. Atoms like 1H,
13C, or 15N, have nuclear spin and give rise to a sharp NMR
signal, whereas atoms like 12C, and 16O have no nuclear spin
and therefore no signal. This makes the technique of NMR very
powerful because different spectra can be taken with molecules
that have been selectively isotopically labeled with atoms
that have or do not have spin.
In an NMR experiment, a sample in a long tube is inserted
into the magnet, and the resonant responses of the atoms in
the sample over a range of frequencies is recorded. These
responses are influenced by the shape of the molecule in which
the atoms resideby their proximity to other atoms in
the molecule. An NMR spectrum is unique for a particular molecule,
and the structure of a molecule can be determined from its
spectrum.
NMR is more than simply a useful tool for chemists and biologists.
It is ubiquitous at universities, biotech companies, and biomedical
research institutes. TSRI is a leader in high-powered NMR
instrumentation, with over 13 instruments above 500 MHz.
The 900 MHz spectrometer is the latest of a large number
of instruments, but there is no doubt that, for a time, it
will be the greatesthere or anywhere. "The fact that
[the 900] is coming to TSRI is a big deal," says Wright, "because
it allows us to push the frontiers of NMR research on biomolecules."
1 | 2 |
|
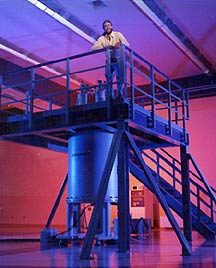
"What TROSY lets you do is to work on really big systems,"
says Molecular Biology Professor Jamie Williamson, seen here
atop the platform for the 800 MHz instrument.
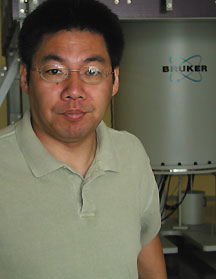
Director
of TSRI's Biomolecular NMR Facility John Chung poses in front
of the 800 MHz.
For More
Information:
Wright/Dyson
Group
Williamson Laboratory
|