(page 2 of 2)
One would take out the patients bone marrow, remove
the stem cells and infect them with the intrabody gene using
the HIV vector, then return the cells to the patient. The
stem cells would then develop into dendritic cells and blood
cells, including cells that HIV infects, such as macrophages
and T-cells. These new cells would be phenotypically equivalent
to CCR5 negative cells since the intrabody would prevent the
coreceptors from reaching the cellular surfaces.
These progeny cells, then, would be effectively resistant
to HIV. Having a selective advantage over the wild type cells,
they would repopulate the body. The intrabodies would then
do what antiretroviral drugs have done for years: keep the
virus in check. "Its no different from protease, really,"
says Torbett. "The idea is to keep the viral level low, protect
the T-cells, and allow the immune system to do its job and
control the infection."
There are many basic questions that need to be addressed
before this type of therapy is ready for human trials, though.
One needs to understand how efficiently the gene inserts and
the intrabody expresses, what regulation machinery is involved,
and how well the intrabody protects the cell. Another unanswered
question is just how fast the virus will mutate to adapt to
the intrabody treatment, just as it does to defeat drugs like
AZT and protease inhibitors. "These concerns are critical
for the success of gene therapy in general and for an HIV
treatment," says Torbett. "My group and our colleagues, Carlos
Barbas and Daniel Salomon, are currently working on these
areas."
Fighting the Virus Without Drug Holidays
One of the major problems with current HIV antiretroviral
therapy is adherence to the drug regimens that are very demanding.
There are so many pills to keep track ofthose to take
with food, and those on an empty stomach; some once a day,
others throughout the dayand some patients just cannot
maintain a constant dosage.
Other patients on therapy go through healthy periods, and
some patients consciously take "drug holidays" to reward themselves
for a special occasion they would like to enjoy while free
from the toxicity of their medicine.
Once they stop, the virus may rebound. Worse, when it comes
back, it may have become a mutated form that is highly resistant
to various drugs. This gene therapy approach could be used
to create a stable immune response without having to take
a rigorous daily regiment of drugs. Instead of an oral compoundor,
more realistically, a combination of compoundsthe intrabody
would be given to a patient once.
Although this approach could one day be used as a vaccine
to protect uninfected people against infection, the picture
here is much more cloudy.
Vaccines are the best hope for controlling HIV in parts
of the world where the epidemic is the most problematic, such
as sub-Saharan Africa and parts of Asia. But besides the efficacy
question for gene delivery, there are unresolved basic science
questions of how to regulate the genes placed into a cell,
and how to keep the immune response from reacting to products
made by the new genes. Those issues notwithstanding, nobody
knows if a treatment like this would be feasible as a traditional
vaccine.
"Its a big if," says Torbett. "Right now,
given the cost, this kind of treatment would be beyond the
scope of many parts of the world. But it could very well protect
people from being infected."
Epilogue: A Technique to Treat HIV and Cancer Both
Inserting HIV intrabody genes is only one of several applications
of Torbetts work to control cellular function. Another
promising application is the treatment of a certain type of
cancer called acute myeloid leukemia (AML), a common form
of acute leukemia in adults.
Myeloid cells, which constitute about 60 percent of bone
marrow cells, are the non-differentiated progenitors of such
diverse blood cells as monocytes, neutrophils, and platelets.
Myeloid cells, in turn, derive from hematopoietic stem cells
during hematopoiesis; a process that relies on a properly
functioning transcription factor called PU.1.
PU.1 is a small, winged helix-turn-helix protein that binds
to a purine rich sequence containing the GGAA motif of DNA.
This protein is a major player in normal or abnormal myeloid
development because it regulates transcription of those genes
that control the lineage and differentiation of myeloid cellsones
expressing cytokine receptors and their signaling pathways,
adhesion molecules, and other key cellular proteins. Knocking
out PU.1 halts myeloid development but does not halt cell
division, a state that may contribute to development of AML.
Aberrant or loss of expression of key genes controlled by
PU.1, such as those that code for cell adhesion molecules
and cytokine receptor signaling pathways, may result in cells
that do not finish their development, yet still can grow,
allowing additional molecular changes that may promote cancer.
The malignant population of immature cells derived from a
single malfunctioning myeloid founder cell may promote AML.
Torbett is looking at ways of controlling AML by controlling
PU.1 transcription. He uses in vivo models that mimic
what happens in normal myeloid development in humans and he
manipulates PU.1 to gain insight into normal and pathological
states and to understand gene regulation. "Our group is looking
at this gene as a paradigm for hematopoietic and myeloid development,"
he says.
Medicines in the future may move away from targeting gene
products with some compounds to turning on and off the genes
involved in cell functions that are relevant to health and
disease. These gene-based drugs could be compounds that mimic
regulatory proteins or even pieces of DNA that, once inserted
into cells, would control regulatory regions.
"The current drugs are useful," he says, "but the future
of molecular medicine comes down to understanding and controlling
the regulation of genes and their products."
1 | 2 |
|
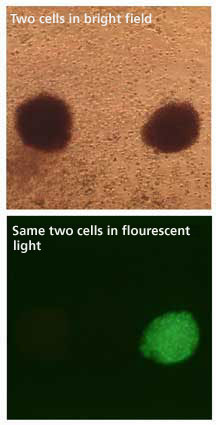
Human cells developed from stem cells
transduced with HIV vectors contain a surrogate marker. In
this case, a green fluorescent protein gives a qualitative
measurement of expression. The cell on the right flouresces,
the one on the left does not. "You can easily distinguish
between cells that have the gene of interest and those that
dont," says Torbett.
|